Guan-En Graham is determined to find out exactly what happened to her father. When she was a child, he developed brain cancer. Since then, she has worked to understand the intricate genetic mechanisms that trigger brain diseases so that one day, perhaps, she can shut them down for good.
Now Graham might have the tool to do it. In the lab of Jeremy Day, Ph.D., the sophomore neuroscience major from Las Vegas is part of a UAB research team investigating CRISPR, a piece of gene-editing technology that could have the potential to prevent disease before patients start to suffer.
Precise and programmable
CRISPR, or Clustered Regularly Interspaced Short Palindromic Repeats, is essentially a pair of molecule-sized programmable scissors—scissors that work on the DNA inside living cells. In the few years since scientists have refined the technology, CRISPR has revolutionized gene editing.Jeremy Day (center) and undergraduate students Guan-En Graham (left) and Jasmin Revanna (right) use CRISPR technology to illuminate the power and reach of epigenetic modifications in brain diseases.
The concept of adding, deleting, or otherwise altering an existing DNA sequence is nothing new; scientists have done it in laboratories for decades. But earlier gene-editing technologies can produce “off-target” effects, unintentionally tinkering with other pieces of DNA. CRISPR is more precise, efficient, versatile, and affordable than its predecessors, which has changed how scientists address questions and the speed in which they answer them.
Day, a UAB School of Medicine assistant professor of neurobiology, studies epigenetics, a group of molecular modifications that influence gene activity without changing the DNA sequence. CRISPR enables his research group “to explore how epigenetic modifications affect expression of specific genes. The ability to express genes in a selective fashion gives rise to the amazing diversity of cell types that we possess.”
Jasmin Revanna, another neuroscience major working with Day, describes the work as “changing ‘tags’ on DNA that affect its function in the cell.” The goal is to discover the roles those tags, or epigenetic modifications, play in diseases that don’t result from DNA mutations, adds the freshman from Jacksonville, Alabama.
“We are applying this to addiction-related diseases to understand how drugs of abuse engage the epigenome to generate long-term alterations in neuron function and behavior,” Day says. The findings could lay the groundwork for a variety of CRISPR-related epigenetic treatments—perhaps boosting or restoring memories for Alzheimer’s patients or tamping them down for people with post-traumatic stress disorder. “While our current studies are in their infancy, this technology is a huge leap forward,” Day says.
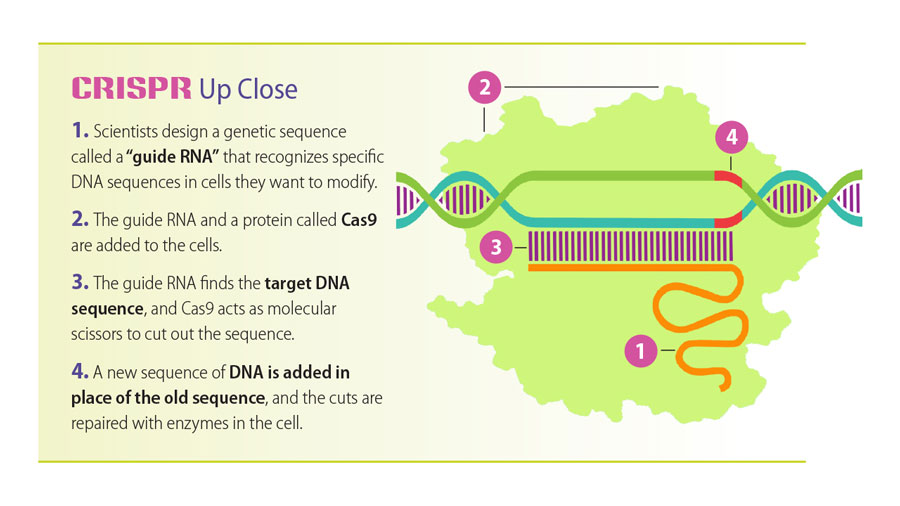
Cut, paste, treat
CRISPR originated in certain bacteria, where it functions as protection against invading viruses. Essentially, it’s a “highly efficient and specific” cellular immune system, explains Tim Townes, Ph.D., professor of biochemistry and molecular genetics in the UAB School of Medicine. The molecular mechanism recognizes viral DNA and cuts it up to prevent the virus from replicating inside the bacteria.
Where CRISPR cuts DNA, researchers can delete or insert a DNA sequence. Townes’s lab has applied this technology to successfully correct the genetic mutation causing sickle cell disease (SCD), which can cause chronic pain and organ damage. Current SCD treatments are lifelong and can have severe side effects.
“All patients with SCD have the exact same mutation in the exact same location on the exact same gene—meaning we need to correct only one mutation in the bone marrow cells to cure someone of this condition forever,” Townes says. The process involves retrieving bone marrow cells from the patient, treating the genes, and then implanting the cells back into the patient. Because the cells are the patient’s own, there would be no need for a transplant or risk of rejection. “With CRISPR we have an effective rate of correction for the sickle cell mutation between 30 and 50 percent. Just that portion of cells is enough to cure someone of sickle cell,” Townes says.
Currently, each baby born in the United States is tested for the sickle mutation, allowing researchers to know within a month whether the baby will develop the disease. SCD symptoms typically do not appear until children are a year old, “which leaves 11 months to cure them, and they may never have to experience the disease,” Townes says. A similar approach could benefit patients with other diseases. In published research, Townes and his team have shown how they used CRISPR to correct the DNA that triggers severe combined immunodeficiency disease, in which children are born with no immune response.
A key to personalized medicine
Both Townes and Day expect human clinical trials of CRISPR-based therapeutics to begin in the next few years. Genetic disorders arising from single-gene mutations, like SCD, are ideal CRISPR candidates because they require only one small correction to be made, and the correction is always the same. However, most genetic disorders aren’t so simple, involving complex mutations in multiple genes. As CRISPR technology improves, it may be adaptable for a wider range of disorders, and both Townes and Day predict that it will play a key role in the development of personalized medicine, with treatments tailored to each patient’s genetic makeup.
Researchers led by Tim Townes have used CRISPR (illustrated above) to correct the genetic mutation causing sickle cell disease — a discovery that points to a potential cure.
Babies born in Alabama hospitals currently are tested for 35 diseases, “but for about the same price, we could sequence their genome” and pinpoint genes that put them at risk for disease, Townes says. Then, “long before someone develops one of these genetic predispositions, we could correct the gene so that they never experience that disease,” Townes says.
Even when gene editing is difficult or impossible, CRISPR’s ability to introduce desirable epigenetic modifications may become highly useful, Day adds. "This will be possible in several years as we develop our basic understanding of how epigenetic marks regulate single genes."
Safety monitors
Could CRISPR lead science down a slippery slope, with people wanting to alter their genes—or their children’s genes—for cosmetic reasons, for example? Safety and ethical considerations are key to CRISPR’s progress, Day and Townes say. “We already use many different therapeutics that are capable of altering how our genes are expressed and capable of producing long-term effects,” Day says. “The ethical considerations that arise have more to do with the application of technology than with the technology itself. Ultimately, the benefits of CRISPR will outweigh potential risks, provided there is adequate screening and careful observation.”
Townes agrees, noting that “overall the scientific community has done well in regulating these new technologies and not acting irresponsibly.”
The Food and Drug Administration, which must approve any clinical trial of CRISPR in humans, also is developing regulations on safety and the use of gene-editing technology.