UAB Researchers Build the Nanoscale Future
By Suzanne Parker
UAB physics professors Yogesh Vohra, left, and S. Aaron Catledge work with chemistry professor Eugenia Kharlampieva in the UAB Center for Nanoscale Materials and Biointegration.The poet William Blake once imagined seeing “a world in a grain of sand,” but Yogesh Vohra, Ph.D., sees a world of possibilities on a much smaller scale. Vohra, a UAB physics professor and director of UAB’s Center for Nanoscale Materials and Biointegration (CNMB), is leading an interdisciplinary team of researchers who are working toward synthesis and characterization of nanoscale materials and structures and subsequent integration of these nanomaterials and nanostructures into practical biomedical devices and technologies.
These scientists, engineers, and physicians are building and manipulating extremely tiny structures that could make a big impact on patient care, from improving drug delivery to developing better implants for joints and blood vessels—and even boosting the success of transplants. But they will never see their handiwork with their own eyes because they’re operating on the scale of atoms and molecules.
Nanoscale, nanotechnology, and nanoscience—all derive their meaning from the Greek word nanos, meaning “dwarf.” A nanometer is a billionth of a meter, and nanoscale structures are constrained in at least one dimension to less than 100 nanometers. By comparison, a grain of sand is 500,000 nanometers, 10 times wider than a human hair, which is approximately 50,000 nanometers. A single red blood cell is approximately 9,000 nanometers.
But there is more to nanotechnology than size. Working at the nano level has forced researchers to redefine their understanding of matter itself. Nanomaterials possess novel physical, structural, chemical, and biological properties and behaviors. For instance, they have a much larger surface area in relation to their mass compared to bigger particles. That means they respond to electricity and magnetic fields, for example, in ways that are only beginning to be revealed as scientists delve into this miniature realm.
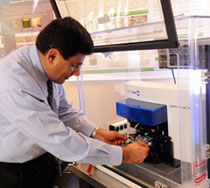
Interdisciplinary Efforts
It takes a large team to think small. “The CNMB bridges the gap between research laboratories, development, and eventual medical applications of nanoscale materials,” Vohra says. For example, a physicist or chemist may conceptualize an innovative nanoscale scaffolding structure for use in vascular grafts to repair compromised blood vessels. Input from clinical researchers and engineers guides the choice of materials that can best attract and encourage cell deposition within the vessels. Then UAB clinicians enter the picture, helping to transform this theoretical concept into a medical device that can be evaluated in human trials. Under Vohra’s leadership, CNMB interdisciplinary research efforts got a boost with a center grant from the National Institutes of Health to hire two tenure-track faculty members in nanoscale sciences for biomedical research: S. Aaron Catledge, Ph.D., in the Department of Physics and Eugenia Kharlampieva, Ph.D., in the Department of Chemistry.
Catledge focuses on the architectural design and construction of nanomaterials—including nanodiamonds. Specifically, he focuses on creating patterns of nanodiamonds on the surface of artificial joints, using patented diamond coatings developed by Vohra’s lab. The result could be orthopedic and dental implants that are more durable than current versions.
Mountains out of Molecules
Just like skyscrapers, nanoscale materials rely on a strong foundation. Catledge uses a process called dip-pen nanolithography (DPN) to build and craft nanostructures. The DPN instrument consists of an atomic force microscope with a probe on the end—the pen—that dispenses biomolecular “inks.” By alternating these inks in successive layers of patterns, researchers can strategically deposit everything from proteins to DNA, assembling increasingly complex structures.
This “bottom-up processing” could help scientists create customized nanoscale landscapes to influence protein function, cellular organization, and tissue regeneration, Catledge says. He says nanoscale scaffolds could provide structure and direction for heart muscle cells to repair damaged heart tissue—or “biosensing” particles that could be engineered to detect the presence of toxic chemicals, cellular antigens, or disease precursors. Integrated into stents or other therapeutic products, these particles could provide an early warning signal of impending trouble.
Nanotechnology has the potential to influence protein production, cellular organization, tissue regeneration, and more.Biological Flattery
What’s the best way to design a micromolecule? Eugenia Kharlampieva, a polymer chemist in the CNMB, finds inspiration in nature—particularly the structure of polymers, the biological building blocks that form proteins, peptides, starches, and nucleic acids. Nanoscale engineering enables her to create synthetic polymers and biomimetic materials, which copy nature’s examples and also improve upon them by controlling their solubility and other properties.
Kharlampieva has worked with silkworm silk, adding metal nanoparticles to silk films to strengthen them for medical applications. Today, she is designing biomimetic polymer coatings that will interact with cell surfaces, which could aid drug delivery, sharpen biomedical imaging, and strengthen the design of dental, joint, and tissue implants.
The coatings, Kharlampieva says, form microcapsules that can transport drugs into cells quickly and easily. “In response to changes in pH or temperature, these smart drugs will open and release contents on demand,” she explains. But the coatings can also protect cells for transplantation. Veronika Kozlovskaya, Ph.D., a postdoctoral associate in Kharlampieva’s group, is working with Anthony Thompson, Ph.D., director of UAB’s Division of Transplantation Research and Development, to encapsulate insulin-producing pancreatic islet cells, a potential treatment for diabetes. Because donor islets must be cultured prior to transplantation, they can disintegrate or fuse together and die. “Our design challenge,” Kharlampieva says, “is to create a coating—like a nylon stocking—to protect the islet’s integrity.” Success could help save lives, one nanoparticle at a time.